Aerospace Micro-Lesson #46
In This Section
Atmospheric Pressure
We live our lives in a sea of air; it literally presses on us from all directions. It is such a part of our existence, in fact, that we tend to take it for granted. It was only in the 1600s that scientists started to figure out that air has pressure at all. Nowadays air pressure is very well understood, from the variations that signal changes in the weather to the variations with altitude that drive the choice of commercial flight routes to the air pressure of artificial atmospheres in manned spacecraft.
Next Generation Science Standards (NGSS):
- Discipline: Physical Science
- Crosscutting Concept: Energy and Matter: Flows, Cycles, and Conservation
- Science & Engineering Practice: Constructing Explanations (for science) and Designing Solutions (for engineering)
GRADES K–2
NGSS: Earth's Systems: Use and share observations of local weather conditions to describe patterns over time.
Hold out your hand. Imagine—or measure—a one-inch square on the palm of your hand. Now imagine a column of air, one inch by one inch, stretching from your hand up and up and up, through the ceiling, through the roof, up through the atmosphere into space. All the air in that column weighs a little less than fifteen pounds.
If you think this sounds familiar, you are right. We speak of air pressure as being a little less than fifteen pounds per square inch. Relative to this, weather forecasters often talk about high pressure and low pressure. Usually, good weather is linked with high pressure and storms seem to come along with low pressure. Air pressure can also change if you visit a different location. For instance, if you climbed to the top of Mt. Everest, the pressure would be a little less than five pounds on a square inch of your hand.
Suggested Activity: Place a flat stick such as a ruler or a yardstick on the edge of a table so that one end and about half its length are hanging over the edge. Place a sheet of newspaper flat over the half of the stick that is on the table with the edge of the newspaper at the edge of the table. Smooth the newspaper down so that there is no space (or air) between the newspaper and the table. If you press down on the free end of the stick you will find that it does not move because the force of the air is pushing down on the newspaper covering the other end. In fact, if you press down hard enough and quickly enough (before the air has a chance to flow in under the newspaper), the stick will break rather than the other end moving up.
NGSS: Matter and its Interactions: Develop a model to describe that matter is made of particles too small to be seen.
Air pressure is measured by a scientific instrument called a barometer. Weather stations often come with a barometer as one of their devices (along with a thermometer, a weather vane, etc.). Before experiments proved that air pressure existed, it was believed that air was weightless. Famous names such as Galileo, Pascal, and Goethe are all associated with the theory of air pressure and/or the development of barometers. Barometers were first developed in the 1640s. They have taken many forms and used many different substances. Some are water-based, some use mercury, and aneroid barometers use a chamber emptied of air and a spring to keep it from collapsing. There is even a type of barometer that records the air pressure on a roll of graph paper, earning it the name barograph. Many aircraft have a barometer-based altimeter as part of their instrument panel. An altimeter indicates the altitude of the craft using the air pressure to identify the distance above sea level.
The lower air pressure at high altitudes affects how high airplanes are designed to fly. Less air pressure means less lift and drag forces on airplanes; many airplanes, therefore, are designed to fly as high as possible. The lift and drag forces also increase with speed so an airplane flying faster at a high altitude will have the same lift and drag forces acting on it as an airplane flying more slowly at a low altitude. The lift needs to be just enough to equal the weight of the airplane so that it doesn’t rise or fall but continues to fly in a straight line. This means that an airplane flying at a higher altitude can fly faster than an airplane flying at a lower altitude and thus get to its destination more quickly.
Suggested Activity: You might like to assemble your own barometer. Directions for one such project are available at Easy Science for Kids.
GRADES 6–8
NGSS: Matter and its Interactions: Develop a model that predicts and describes changes in particle motion, temperature, and state of a pure substance when thermal energy is added or removed.
Air presses in on you on all sides and in all directions. Fortunately, your body presses out with exactly as much pressure so that the pressures balance and you do not get squeezed.
When a rocket is launched into space, the air pressure surrounding it drops from normal atmospheric pressure down to essentially zero over the course of a few minutes. Without the air outside the rocket pressing in on it, any air inside the rocket will still push out and there will be an imbalance in pressure. Either the skin of the rocket must be strong enough to handle the pressure imbalance, or the rocket will distend under the pressure, or there must be a way to let the air out quickly enough that the pressure cannot build up. The first alternative, making the rocket strong enough to withstand the imbalance, is not good; strength means weight and extra weight is bad. Letting the rocket skin distend under the pressure is also unacceptable. The only acceptable alternative is to make sure that every compartment on the rocket has a way to let the air out. (Skylab, the first American space station, was almost lost because of poor venting; an unvented compartment expanded and jammed one of the solar panels so that it could not open.) NASA has an entire office devoted to making sure that all the compartments on spacecraft are properly vented.
People speak of “the vacuum of space,” and space is indeed a vacuum. This does not mean that it is completely empty, though. There is no physical line below which one is in the atmosphere and above which one is in a perfect vacuum; instead, the density of the air gets smaller and smaller until it can be neglected. (How low a density can be neglected depends on what one is trying to do; the air density that one can neglect when calculating a single orbit of a spacecraft will eventually cause that orbit to decay and the spacecraft to re-enter the Earth’s atmosphere.) At an altitude of 300 kilometers, for example, the “air” has a density of about 2.4×10-11Kg/m3 , which translates into about 8×1014 atoms or molecules per cubic meter. (By way of comparison, at sea level the atmosphere contains about 2.6×1022particles per cubic meter.) Farther out, at the altitude of geostationary satellites, the density has decreased to that of the interplanetary medium, which is about 5,000,000 particles per cubic meter near the Earth. For visualization purposes, it may be better to express this last value as about five molecules per cubic centimeter.
Suggested Activity: If you have a way to heat something in the classroom, get a metal container such as cooking oil comes in. (The school cafeteria is likely to have one.) Put about an inch of water in the bottom and put the lid on loosely. Now heat the container so that the water boils; the steam will push out much of the air. After the water has boiled for a minute or so, tighten the lid (using oven mitts or potholders!) and turn off the heat. As the steam condenses back into liquid water, the pressure inside the container is less than the pressure outside. The air pressure will make the container collapse.
GRADES 9–12
Air pressure is caused by the molecules of air’s components bouncing off a surface. If you will imagine a single “air molecule” like a rubber ball inside a box bouncing off the sides, each time the molecule bounces off the sides it adds a little to the pressure. Let us consider a molecule with mass “m” moving with an x-direction speed “Sx” in a cubic box of side length “L”.
Pressure is defined as force per unit area; force is the rate of change of momentum; momentum is the product of the mass and the velocity. The molecule’s momentum changes from “+mSx” to “–mSx” every time it bounces off the +x side of the box. The time between bounces off the +x side of the box is the distance traveled divided by the speed, or “2L/Sx”. The force is therefore given by the formula:

The pressure is the force per unit area; the area of the +xside of the box is simply L2. The formula for pressure is therefore.
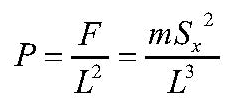
The volume “V” of the box is just the cube of the side’s length “L”. We can rearrange our equation for the pressure to read

This is simply Boyle’s Law, which we learn in high school physics class: the product of the pressure and the volume of a gas is constant for a constant temperature. One can derive Charles’ Law, which states that the ratio of the volume to the absolute temperature of a gas is constant at constant pressure, by pointing out that the absolute temperature is proportional to the square of the molecule’s speed.
This is an extremely simplified derivation of Boyle’s Law. The rigorous derivation requires triple integrals (high-powered calculus) over a six-dimensional phase space (three position coordinates and three velocity coordinates) and gives pretty much the same result.
Sixty Years Ago in the Space Race:
September 7: The Soviets launched a second R-7 rocket to an altitude of 840 miles.