Aerospace Micro-Lesson #92
In This Section
Luna 3: Seeing the Far Side of the Moon
Sixty years ago this month, the Soviet Luna 3 space probe flew past the Moon and photographed its far side. Nobody, from the beginning of the human race until that time, had ever seen what the back side of the Moon looked like. The grainy, low-resolution photographs showed a surface very much unlike the front side. This lesson describes the mission and what it found.
Next Generation Science Standards (NGSS):
- Discipline: Engineering Design
- Crosscutting Concept: Systems and System Models
- Science & Engineering Practice: Constructing Explanations and Designing Solutions
GRADES K–2
If you’ve invented a rocket that can send something into space, what do you do with it? One answer is that you start exploring space and the other worlds around you, places that nobody has ever been before. That is what the Russians did sixty years ago after they had put the first satellite into orbit around the Earth. Their first satellite, Sputnik 1, was launched in October 1957; a year and a half later, they were trying to launch spacecraft to explore the Moon.
They didn’t succeed right away. In fact, the first mission to successfully send back photos of the far side of the moon was the seventh attempt at a lunar probe by the Soviets. Besides its two Luna predecessors, there were also two probes that literally shook themselves apart because their launch rockets vibrated so much, a third that was underpowered because a seal leaked, and a fourth that went off course and failed to reach Earth orbit.
Luna 1, seen in the first picture, was launched in January 1959 carrying instruments to study magnetic fields, cosmic radiation, and interplanetary matter. It was supposed to smash into the Moon, but there were problems with its guidance systems and it flew past the Moon at a distance of 5,995 km (3,725 miles). Luna 1 was the first object created by humans to escape the pull of the Earth’s gravity and travel to the moon and beyond. (How do you miss a target that is two thousand miles wide? You aim at it from 239,000 miles away.)
Luna 2 was the first artificial object to reach the surface of another celestial body. It carried the same instrument package as Luna 1. About halfway to the Moon, it released a cloud of sodium gas out into space. The sodium gas it released was observable from the ground, helping scientists to see how gas behaves in space. Along with its science gear, Luna 2 also carried metal spheres which were designed to burst apart and spread pentagonal “pennants” (seen to the right) across the lunar surface.
The next step in the Soviet exploration of the Moon was to send a space probe behind it, to take pictures of the back side of the Moon and send them back to Earth. To understand how important this was, you have to understand that the Moon always keeps the same side facing the Earth. If you go outside in the evening and look at the Moon, you will always see the same side. With the different phases of the Moon, you may see different parts of this side, but you never see the back side of the Moon. In fact, nobody on Earth had ever seen the back side of the Moon, ever. Nobody knew what it looked like.
Sixty years ago, on October 4, 1959, the Soviets launched their Luna 3 mission to fly around behind the Moon and photograph its back side. Three days later, Luna 3 reached the Moon. It took 29 pictures with an old-fashioned camera that used film (it was a very modern camera in its day), developed the film onboard the spacecraft, and then transmitted the image on the film point by point back to Earth. Of the 29 pictures, 17 showed something that people could understand as part of the back side of the Moon. After it transmitted its pictures, its mission was accomplished. It re-entered the Earth’s atmosphere some time between 1960 and 1962.
GRADES 3–5
Sixty years ago this month, the Soviet Luna 3 spacecraft took the first photographs of the far side of the Moon and sent them back to Earth. Before then, nobody had any idea what the back side of the Moon looked like. Most scientists thought that it looked a lot like the front side, with large dark smooth “maria” surrounded by craters and mountains. (There was even a running joke at the time that the Moon itself was made of green cheese.)
Those first images were very grainy and lacked much of the fine details that we can see today. NASA has a side-by-side comparison of the Luna 3 image and a composite of pictures taken by the Lunar Reconnaissance Orbiter (LRO). Looking at the two pictures (which you can also see on the next page), it is easy to see the vast improvement in imaging technology during the 50 years between the two missions. The two pictures on the next page are side by side; the link goes to a picture with a slider which you can slide back and forth, showing the Luna 3 and the LRO images together. (It may be appropriate here to note that it is for pioneers to be the first, not the best. This may also be a good point to ask the students what they think technology will be doing sixty years from now, as far into the future as Luna 3 is in the past.)
NASA’s Astronomy Picture of the Day has one of Luna 3’s pictures before it was stitched together with the other pictures to give the view of the complete disk. Other pictures from Soviet Moon probes can be found here.
When one is the first person to find something, one usually has the right to name it. The Soviets took advantage of this right when they started naming features on the back side of the Moon. Many far-side features are named after places in Russia (like the “Sea of Moscow”) or, more frequently, scientists or Soviet heroes (like Gagarin, Korolev, and Tsiolkovskiy Craters). They did not discover everything on the far side, though, so we also have craters named Apollo (for the American project), Pasteur, Leibnitz, and so on. The larger features on the near side of the Moon, on the other hand, were named by astronomers a few hundred years ago. The first people to assign most of the currently-used names, two astronomers named Giovanni Riccioli and Francesco Grimaldi, divided the near side of the Moon into eight segments and assigned names to craters in each segment according to a chronological rule. The first three segments had names from antiquity—ancient astronomers and figures from mythology. The segments proceeded through history to the eighth segment, which contained names from Riccioli’s and Grimaldi’s contemporaries in the mid-1600s. They named the lunar “seas” after states or things that they thought a sea would have—tranquillity, storms, waves, and so on.
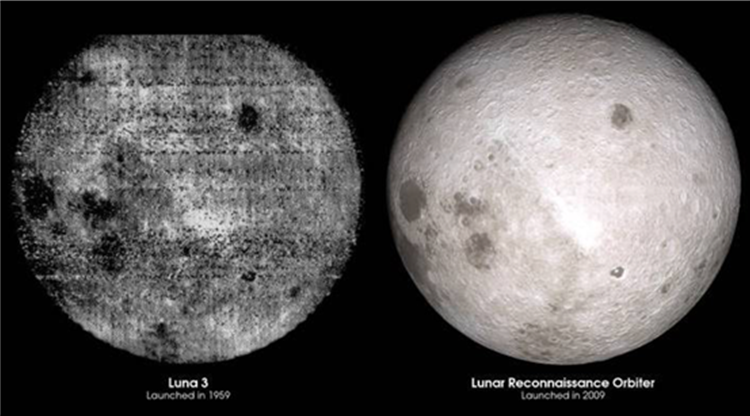
GRADES 6-8
MS-ETS1-1. Define the criteria and constraints of a design problem with sufficient precision to ensure a successful solution, taking into account relevant scientific principles and potential impacts on people and the natural environment that may limit possible solutions.When Luna 3 was launched, engineers were still figuring out how to navigate in space. Their first attempt to launch a spacecraft to hit the Moon, called Luna 1, missed by over 3,000 miles. (Lest this reflect poorly on Soviet space navigation, we note that the American Pioneer 4 mission, launched a few weeks after Luna 1, missed its intended fly-by distance by about 18,000 miles.)
How do you navigate in space? There are two parts to the problem. The first part is knowing where you are; the second part is knowing how to get to where you want to be.
Figuring out where you are on Earth depends first of all on landmarks. You measure the direction to one recognized object, you measure the direction to another recognized object, you draw lines on the map from those two objects back from the directions you measured, and where the lines cross is where you are. This is called a navigational “fix.” For more accuracy, you use three objects and draw three lines. (When the three lines don’t all come together at a point, but instead form a small triangle, that gives you some idea of how much error there is in your measurements.)
In space, you are navigating in three dimensions so you would need at least threemeasurements of direction to three objects to get your “fix.” Unfortunately, unless you are in orbit around something you don’t generally have three objects that are close enough for you to measure the angles. No angle measurement is perfect, and the position error that arises from an error in an angle measurement increases in proportion to the distance from the object being measured. If you measured the direction to the planet Venus, for example, which never gets closer to the Earth than about 25,000,000 miles, a measurement error of 0.01 degrees in your angle translates into a position error of around 4,500 miles.
Modern navigation on the Earth is often done using the Global Positioning System. This consists of 30 satellites in Earth orbit broadcasting their time and position on a continuous basis. A GPS receiver receives these broadcasts and from the relative arrival times of the signals from the different satellites figures out its distance from those satellites and thus its position. Unfortunately, absent a Universal Positioning System in space, this does not work outside of Earth orbit.
Navigation in space is most frequently done by what is called “dead reckoning.” (The term comes from the days of sailing ships, when the navigator would calculate his position from the last “fix” based on the ship’s speed and direction. There is a controversy over the origin of the term after that.) In dead reckoning, you measure your initial position and velocity and then calculate the later positions by using the fact that your velocity is the rate that your speed changes over time. Space flight is uniquely suited to dead reckoning: apart from isolated rocket motor burns (which you theoretically control), the only significant forces on your spacecraft are gravitational forces which you can calculate very accurately.
Helping with dead reckoning is that a ground station on the Earth can measure the radial speed of a spacecraft—the rate at which the spacecraft’s distance from the ground station is increasing or decreasing—very accurately. Angle measurements from the ground station tend to have rather large errors, but the radial speed measurement is extremely accurate. Not only does this feed directly into a more accurate distance calculation, but comparisons with the expected values based on where a spacecraft is relative to a nearby planet or moon (and specifically how strongly the planet’s or moon’s gravity is attracting the spacecraft) can be used to refine the estimates of exactly where the spacecraft is.
The second part of knowing where you are in space is knowing your orientation—which direction is what. On Earth, it’s pretty obvious which way is up. In space, without the feel of gravity pulling you “down,” it is not so obvious. Outside of a planetary or lunar orbit, the terms “up” and “down” have no meaning. Fortunately, finding your orientation in space is pretty simple: you can sight directions to the stars and orient yourself relative to them.With the knowledge of where you are and which direction you are facing, you can fire your rocket motor to propel yourself to where you want to be. But figuring out where you want to be is not trivial either. You probably want to rendezvous with a planet or other celestial body, and everything in space is in motion. Not only do you need to get to the right place, but you need to get there at exactly the right time. Fortunately we know where all the large Solar System objects are, to within a few meters. (This link shows the distances of the planets and some other Solar System objects from the Sun, down to the nearest kilometer. Impressive as it is, it does not show the full extent of our knowledge.) Your students may be impressed by the fact that this means, for distances of hundreds of millions of kilometers, that the positions are known to a precision of eleven significant figures. Try measuring anything else to eleven significant figures!
But back in the days of Luna 3, nobody knew any of this. Luna 3’s controllers launched it northward, over the Earth’s North Pole, toward the Moon—or rather, toward where the Moon would be in when Luna 3 got there in three days. Luna 3 passed over (or would that be “under”?) the Moon’s South Pole and flew behind the Moon. A photo-detector sensed the light from the Moon shining on it and commanded its cameras to start working. (Can your students figure out what the phase of the Moon should have been as seen from Earth at that time? It was five or six days after the New Moon so that the Moon’s back side was mostly sunlit; it would not have made sense to launch this mission near a Full Moon.) The cameras were fixed to the body of the spacecraft, so the whole spacecraft had to turn to point the cameras in different directions. Coming up over the Moon’s North Pole, the spacecraft was catapulted by the Moon’s gravity back toward the Earth. It transmitted its pictures back to Earth and went into a short-lived orbit around the Earth. There was no rocket motor on board to adjust its orbit and it burned up in Earth’s atmosphere sometime in the next few months or years.
Grade 9-12
When the Luna 3 spacecraft was being designed and built, scientists and engineers were still figuring out the very basics of spacecraft design. There was a lot about the space environment and materials’ responses to that environment that they did not know about.
The primary mission of Luna 3 was photographing the back side of the Moon. Because digital cameras with their CCDs (charge-coupled devices) had not been invented yet, to take a photograph the mission designers needed an old-fashioned camera with photographic film. The spacecraft used a photocell (something to detect bright objects) to trigger the camera to start taking its photographs. The camera was not mounted on a swivel, either, so the entire spacecraft had to turn to make it point in different directions to photograph different parts of the Moon’s back side.
The Soviets also did not have a way to return the exposed film to Earth to be developed at home, so they had to design a “darkroom” (place to develop the photographs) on the spacecraft itself. This “darkroom” housed the necessary chemicals—in a water solution—and developed the photographs after they were taken.
With the photographs “in hand,” the spacecraft still had to transmit them to the Earth. To do this, it used a scanner that shone a tiny dot of light on each point on the photograph, measured how bright the reflected light was, and transmitted that brightness back to Earth. This is why the original photographs were so grainy. The first time the mission controllers commanded the spacecraft to transmit the pictures, the spacecraft was too far from the Earth and the radio signal was too weak for them to read. Later attempts were more successful and some 12 or 17 (there is some confusion to the exact number) photographs were received in usable form.
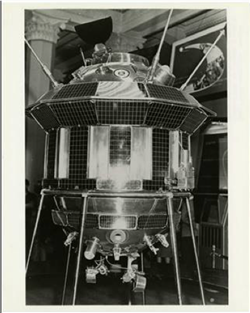
In one of the stranger stories of espionage from the Cold War, it seems that American spies actually “kidnapped” the Luna 3 spacecraft before it was launched, took it apart and examined its various systems to see how they worked, and put it back together and returned it before the Soviets realized it was missing. That said, it also seems that the film that the Soviets used on board Luna 3 to take the photographs of the Moon had been taken from spy balloons that the Americans had flown over Russia in a largely unsuccessful attempt to photograph their secret military bases. (This story may be apocryphal, though.)
Sixty Years Ago in the Space Race:
October 4: The American Mercury space capsule aerodynamics were tested on the "Little Joe 6" test flight.
October 7, 1959: The Soviet Luna 3 spacecraft flew around behind the Moon, giving humanity its first glimpse in all of history of what the Moon's far side looks like.